
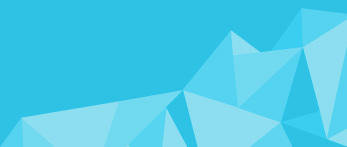
Report on the 18th iPERC Seminar
- 2025/02/19
On July 29, 2024, “Dr. Warren’s Journal Club” was held at the innovative Photonics Evolution Research Center (iPERC) in Shizuoka University. Journal Club is a lecture where students from Shizuoka University and Hamamatsu University School of Medicine read an English paper and discuss the fusion area of engineering, informatics, and medicine. Dr. Warren visited Hamamatsu campus, and the journal club was held as a hybrid of the local and online. The journal club focused on the paper (In vivo imaging with a fast large‐area multiphoton exoscope (FLAME) captures the melanin distribution heterogeneity in human skin, Sci Rep 2022, 12, 8106.). The paper demonstrated the ability to evaluate the 3D distribution of epidermal melanin content in vivo macroscopically (millimeter scale) with microscopic resolution (sub‐micron) and rapid acquisition rates (minutes).
Introduction
Melanin is a group of molecules with multifunctional characteristics and a chromophore responsible for pigmentation of the skin. The prevalent forms of melanin in the skin are eumelanin (brown-black) and pheomelanin (yellow-reddish). They are produced by specialized cells, called melanocytes, mainly found in the basal layer of the skin epidermis. Stored in melanosomes, they are transferred to keratinocytes to protect the skin against photo-damage from UV radiation. Melanin plays a significant role in a variety of physiological and pathological conditions. Reliable measurements of the cutaneous melanin distribution and the melanin-related sensitive dynamic changes are essential for a better understanding and more efficient treatment of pigmentary skin disorders and for differentiating melanoma from benign pigmented lesions.
The current gold standard methods for melanin quantification in skin involve ex vivo*1 chemical analyses. These approaches are invasive and thus, impractical to be performed repeatedly for applications such as those related to pigmentary skin disorders that require monitoring of the treatment response.
At present, methods based on electron paramagnetic resonance (EPR)*2 offer limited spatial resolution (tens of micrometers). Multispectral photoacoustic imaging enables non-invasive 3D mapping of epidermal melanin based on the detection of ultrasonic waves generated by rapid thermoelastic expansion of melanin induced by pulsed laser irradiation. Remarkably, this approach generates images of melanin and blood vessels from 1 to 2 mm below the skin surface, but the 5–30 μm spatial resolution of the images is not sufficient for resolving the epidermal melanin depth profiles.
On the other hand, two-photon excited fluorescence (TPEF)*3 laser-scanning microscopy technologies can generate in vivo 3D sub-micron resolution images of epidermal melanin distribution in human skin. The penetration depth of this imaging technique is limited to 150–200 μm depending on the skin type and other imaging parameters. Nonetheless, this depth is sufficient for capturing the entire thickness of the epidermis on most areas of the body. Enhanced specificity of the melanin detection in the skin is further achieved using two-photon fluorescence lifetime imaging (FLIM)*4 by exploiting the melanin fast fluorescence decay (< 0.2 ns) compared to the fluorescence decay of other fluorophores in the skin.
To enhance the clinical imaging feasibility of this approach, their group and others proposed and demonstrated significant enhancement in image acquisition time based on temporal binning*5 along with analysis of the fluorescence temporal decay slope.
In this work, they employed a fast large-area multiphoton exoscope (FLAME), which is a multiphoton imaging system optimized for clinical skin imaging that has the ability to rapidly generate 3D images (within minutes) over macroscopic areas of skin (up to 1 cm2) with sub-cellular resolution (0.5-1 μm), to evaluate in vivo, in human skin, the significance of the increased imaging area on the epidermal melanin measurement’s reliability.
Results & Discussion
Figure 1(A) shows the macroscopic maps (millimeter scale) for the melanin volume fraction (MVF) as z-projections corresponding to sun-exposed (dorsal forearm) and non-sun-exposed (volar forearm) areas for subjects with Fitzpatrick skin types I–V.*6 These images clearly illustrate the heterogeneity in the melanin distribution, particularly for skin type I.
Figure 1 (A) (B)
Figure 1. (A) The z-projection of MVF for representative subjects with skin types I-V, from the volar and dorsal forearm. Scale bar = 1 mm. (B) 2D-melanin density as a function of epidermal depth from the basal layer (0) to stratum granulosum (1) for all the skin types.
To evaluate the z-distribution of the epidermal melanin content, we measured the average melanin density for each epidermal layer. Here, the position across the epidermis was normalized against the epidermal thickness starting from the basal layer (0) to stratum granulosum (1) (Figure 1B). They generally measured a higher melanin density in the epidermal layers close to the stratum basale compared to the upper epidermal layers. And the global MVF values for the subjects’ dorsal and volar forearms are summarized in Figure 2. The global MVF values increase with skin type and exhibit an overall change of ~ 7 × from skin type I to V.
Figure 2. Figure 3.
Figure 2. The global MVF values for all the skin types from both dorsal and volar forearms. Figure 3. z-projection of the MVF values from the dorsal forearm of representative subjects with skin types I and V, respectively.
The images and data in Figure 3 illustrate the degree of variability of the melanin density along and across the epidermis within representative subjects with light and dark skin types I and V, respectively. As illustrated in Figure 3, the MVF maps measured over macroscopic, mm-scale skin areas include skin folds, hair follicles, and pores. These features are too large for measurements performed over smaller, sub-mm scale scanning areas. These showed the heterogeneity in the 3D distribution of melanin in human skin for all skin types, which further suggests that a large sampling volume is critical for the reliability of the MVF measurements as they would capture this heterogeneity.
As expected, employing the largest FOV (1.6 × 1.6 mm2) would require the smallest sample size for detecting 10% to 25% changes in the MVF values. To detect the same change, the smallest FOV (0.25 × 0.25 mm2) requires ~ 4 to 5 times higher sample size. The fewer samples required when using a larger FOV for imaging could translate to a further decrease in the acquisition time.
Conclusion
In this study, they employ FLAME, a fast, large area multiphoton exoscope, a device with unique performance features optimized for clinical skin imaging, to evaluate the significance of the large volumetric sampling enabled by this instrument on the cutaneous melanin measurements reliability. they demonstrated significant enhancement of the melanin density and distribution measurements reliability across Fitzpatrick skin types I to V based on the ability of this imaging device to capture the intra-subject pigment heterogeneity at the microscopic scale. They expect these advances to have an important impact in clinical and research applications related to monitoring pigment modulation as a response to therapies against pigmentary skin disorders, skin aging, as well as skin cancers.
Q & A
Q1: What is the definition of exoscope?
A1: The precise definition remains uncertain, but it is believed to signify a microscope. It is postulated that this constitutes a more expansive field of view.
Q2: What wavelength?
A2: The specific wavelength was not referenced in the discussion. In the system, infrared was utilized, which may indicate that the wavelength is 780 nm. UV light is for harmful to our skin and it takes some at least 50 minutes for one sequence of this examination.
Q3: Is it possible to improve on that or is it not important at all?
A3: One important thing is we do think the SNR (Signal to Noise Ratio) we need a higher SNR for better analysis. If we can reduce the SNR, we can speed up the measurement. One technique is large area scanning at low resolution and then going to a specific area and doing for higher resolution sparse sampling. The scanner has two different types of scanners as well, one very fast for One Direction and then a little bit slower for larger distance for the others.
Q4: What is interesting about this paper?
A4: The involvement of various disciplines such as physics and biology, as well as medical engineering, in trying to solve problems from different perspectives.
Comments
FLAME, the technology at the center of this paper, combines engineering and medicine to realize more innovative and promising biological imaging.
One of the key points of this technology, the ability to scan a larger area (1×1 mm²) at high speed, is of great engineering interest as it involves laser optics technology as well as electronics and software technology to control it. In addition, non-invasive, real-time observation of biological tissues and the ability to observe deep structures of biological tissues are medically indispensable, leading to early detection of skin diseases and reduction of patient burden.
I believe that the time spent learning about the FLAME technology, which directly reflects the results of engineering and medical developments, has given us a sense of the value of the medical-engineering fusion field and reaffirmed the importance of discussing the further development of this field.
Presenters
Mx. Yuna Tomochika (6th year), Faculty of Medicine, Hamamatsu University School of Medicine
Mx. Ayaka Shimizu (5th year), Faculty of Medicine, Hamamatsu University School of Medicine
Mr. Ryota Akai (2nd year, doctor course), Graduate School of Medical Photonics, Shizuoka University
Mx. Sayuri Takeo (2nd year, doctor course), Graduate School of Medical Photonics, Shizuoka University
Glossary
*1: ex vivo
An analysis in which a test substance is administered to cells or tissues removed from a living body and the reaction is detected.
*2: Electron Paramagnetic Resonance: EPR
A spectroscopic technique in which a sample is irradiated with microwaves and the resonance of electron spins is observed [1]. ESR detects free radicals (unpaired electrons) in a material and provides information about the environment in which the electrons exist. ESR spectra allow us to analyze the presence or absence of unpaired electrons, their quantification, and the state of atoms around unpaired electrons. In principle, ESR is 700 times more sensitive than NMR (Nuclear Magnetic Resonance), which also detects nuclear spin in a magnetic field.
*3: Two-photon excited fluorescence (TPEF) (Figure 4)
A device that uses ultrashort pulsed near-infrared laser light for the imaging the 3-dimensional distribution of fluorescence intensity (concentration of fluorescent molecules) inside living organisms (up to several hundred μm deep) [2]. It enables a huge advantage in biological imaging especially in thick tissues and live cells studies.
Figure 4.
Figure 4. Excited-state energy diagram showing two-photon excitation (1), followed by nonradiative vibrational relaxation (2) and spontaneous fluorescence photon emission (3). In conventional fluorescence detection systems, excitation is achieved by absorption of a single photon of energy (hυEX); processes (2) and (3) are essentially the same.
*4: Fluorescence lifetime imaging microscopy (FLIM)
FLIM measures the time a fluorophore remains in an excited state before emitting a photon and detects molecular variations of fluorophores that are not apparent with spectral techniques alone [3].
*5: Temporal binning
A technique that trades off resolution to reduce noise while increasing the frame rate and dynamic range of the camera. Instead of reading the data from individual pixels, the data from adjacent pixels is combined and read together as a super pixel.
*6: Fitzpatrick skin types
A semi-quantitative scale consisting of six skin types that describe skin color according to basic skin tone, melanin content, inflammatory response to UV light, and carcinogenic risk.
References
[1] ESR/EPR (Electron Spin Resonance) Measurement Service (Contract Analysis)
https://keycom.co.jp/js/sesr/mokuji.html
[2] Principles of Two-Photon Excitation Fluorescence Microscopy, Kohshin Co.
https://optipedia.info/app/lsm/2pfm-principle/
[3] Development of a new technique to analyze the properties of mitochondrial inner membrane by fluorescence lifetime – Discovery of changes in membrane fluidity induced by cellular stress -, Transformative Biomolecular Research Institute, Nagoya University
https://www.itbm.nagoya-u.ac.jp/ja/research/2024/05/post-77.php
innovative Photonics Evolution Research Center (iPERC)
3-5-1 Johoku, Chuo-ku, Hamamatsu, Shizuoka 432-8011 Japan
phone: +81-53-478-3271 / fax: +81-53-478-3256